The Unique Precision: Unraveling the Mechanism of ELISA Through Antibody Antigen Interaction
The exceptional specificity of how antibodies and antigens interact forms the foundation of the reliability and precision of ELISA tests. Antibodies, which are proteins generated by our immune system possess a capability to identify and attach to specific antigens with utmost accuracy [1]. This recognition process is facilitated by the 3D structure of the antibody’s binding site, which perfectly matches the shape and chemical characteristics of the target antigen.
The interaction, between antibodies and antigens is guided by covalent bonds like hydrogen bonds, ionic interactions and van der Waals forces all contributing to creating a stable complex between them [2]. The strength and specificity of this bond is influenced by growth factors such as antibody affinity, antigen concentration, well as environmental conditions like pH, temperature and ionic strength.
The precision in recognizing antibodies, antigens play a role in enhancing ELISAs detection accuracy. By capitalizing on this interaction mechanism ELISA can effectively differentiate molecules while minimizing non-specific bonding tendencies to reduce false positive outcomes [3]. In scenarios precise identification of pathogens, biomarkers or disease specific antigens holds importance for successful treatment and management of illnesses.
Immobilization and Capture: Setting the Stage for the Antibody-Antigen Interaction
In the ELISA process the initial step is to anchor either the antigen or the antibody, onto a base, like a microplate well or membrane. This anchoring paves the way for the following interaction between the antibody and antigen which’s vital for detecting the desired substance.
Many methods are used to immobilize these components, including; adsorption, covalent bonding or affinity capture. Adsorption involves weakly binding the antigen or antibody to the base while covalent bonding forms connections using chemical agents. Affinity capture uses molecules such as streptavidin or protein A/G to trap biotinylated or Fc containing antibodies.
Once either component is anchored in place its corresponding counterpart (antibody or antigen) is introduced to the base allowing for an interaction between them. This phase is called capturing, where the target substance, in the sample binds with its partner to create an antibody antigen complex. How they are positioned can greatly influence how easily the antigen binding sites can be accessed and react ultimately affecting the sensitivity and specificity of the ELISA. It is essential to optimize the immobilization and capturing processes to ensure the assays accuracy and reproducibility are maximized.
The Enzyme-Linked Duo: Amplifying the Signal for Sensitive Detection
While ELISA relies on the interaction between antibodies and antigens the enzyme linked detection mechanism boosts the signal allowing for precise measurements [7]. This enhancement is made possible by introducing an enzyme linked antibody that selectively binds to the antibody antigen complex.
The secondary antibody is paired with an enzyme, peroxidase (HRP) or alkaline phosphatase (AP). These enzymes facilitate the conversion of a non-luminescent substrate into an luminescent end product. The intensity of the resulting color or luminescence corresponds to the concentration of the target substance in the sample [8].
The selection of enzyme combinations depends on factors, such as; desired sensitivity, dynamic range and compatibility with the sample matrix. HRP based systems are commonly preferred for their sensitivity and versatility with substrates like 3,3′,5,5′ tetramethylbenzidine (TMB) or 2,2′ azino bis(3 ethylbenzothiazoline 6 sulfonic acid) (ABTS) [9]. On the hand AP based systems offer enhanced stability. Are ideal, for scenarios requiring minimal background noise. The enzyme linked detection system enhances the amplification, thereby enabling the identification of amounts of the target substance. This makes ELISA a valuable tool, for purposes such as diagnosing diseases analyzing biomarkers monitoring the environment and testing food safety. Furthermore, the precise measurement of the signal allows for quantification of analyte levels offering information, about disease development, treatment effectiveness and biological functions.
Washing Steps: Ensuring Specificity by Removing Non-Specific Interactions
While the specificity of antibody-antigen interactions is inherently high, the presence of non-specific binding can compromise the accuracy of ELISA results. Non-specific binding refers to the undesirable interactions between assay components and the solid support or other molecules present in the sample matrix [11]. These interactions can lead to false-positive signals and inaccurate quantification.
To mitigate the effects of non-specific binding, ELISA protocols incorporate critical washing steps at various stages of the assay. These washing steps involve the removal of unbound or non-specifically bound molecules by subjecting the solid support to multiple rinses with a wash buffer [12].
The composition of the wash buffer plays a crucial role in minimizing non-specific interactions while preserving the stability and activity of the antibody-antigen complexes. Common components of wash buffers include detergents (e.g., Tween-20, Triton X-100) to disrupt hydrophobic interactions, salts (e.g., NaCl, PBS) to modulate ionic interactions, and protein blockers (e.g., BSA, casein) to occupy non-specific binding sites [13].
Proper optimization of the washing conditions, including the number of washes, wash buffer composition, and incubation times, is essential for achieving the desired specificity and sensitivity of the ELISA. Inadequate washing can lead to high background signals and false-positive results, while excessive washing may result in the loss of specifically bound antibody-antigen complexes, reducing the assay’s sensitivity [14].
By effectively removing non-specific interactions through optimized washing steps, ELISA assays can maintain their high specificity, ensuring accurate detection and quantification of the target analyte, even in complex sample matrices.
Analysis of Data; Interpreting the Colorimetric or Luminescent Signal
The last stage of the ELISA process includes analyzing and interpreting the luminescent signal produced by the enzyme reaction. This step translates the interaction, between antibodies and antigens into a result allowing for detection and measurement of the target substance [15].
In ELISA the intensity of the color produced by the enzyme reaction is measured using a spectrophotometer or a microplate reader. The absorbance values obtained are directly related to the concentration of the target substance in the sample. To determine analyte levels accurately a standard curve is created by plotting absorbance values of known analyte concentrations against their concentrations.
On the hand luminescent ELISA relies on measuring emission generated by enzyme catalyzed chemiluminescent or fluorescent reactions. Specialized luminometers or microplate readers with luminescence detection capabilities are utilized to measure this luminescent signal. Similar to ELISA a standard curve is established to correlate luminescence values, with analyte concentrations [16].
Data analysis and interpretation play critical roles in ELISA, as they involve converting raw signal data into quantitative measurements. Different methods are available for data analysis data for example, curve fitting algorithms, background subtraction, and statistical analyses are used to guarantee dependable and consistent outcomes [17].
Additionally, factors such as the dynamic range, sensitivity, and specificity of the ELISA assay must be considered when interpreting the data. Proper validation and quality control measures are essential to ensure the accuracy and reliability of the quantitative results obtained from ELISA [18].
Versatility in Applications: Tailoring ELISA for Diverse Analyte Detection
The adaptability of ELISA is highlighted in its capacity to be customized and adjusted for the identification and measurement of a spectrum of substances including proteins, hormones, antibodies, small molecules and even entire cells or viruses [19].
Within diagnostics ELISA is widely employed for detecting disease biomarkers, infectious agents and autoantibodies. Through the use of antibodies or antigens ELISA can precisely. Measure the presence of disease linked molecules in patient samples aiding in early detection as well as disease and treatment monitoring.
In the realm of development ELISA plays a role across various phases ranging from validating targets and screening lead compounds to conducting pharmacokinetic and immunogenicity studies.
In the realm of research ELISA plays a role in quantifying signaling molecules, growth factors, cytokines and various biomolecules that are integral to different biological processes. ELISAs adaptability lies in its capacity to create antibodies or antigens tailored for nearly any target molecule. By selecting and optimizing these pairs ELISA can accurately measure a wide range of analytes with precision.
Furthermore, the modular design of ELISA permits the integration of enhancements like chemiluminescent or fluorescent detection systems, multiplexing capabilities and miniaturization, for high throughput analysis or point of care testing. In general, the interaction, between antibodies and antigens in ELISA along with its flexibility and versatility has established it as an instrument, across domains.
References
- Delves, P. J., Martin, S. J., Burton, D. R., & Roitt, I. M. (2017). Roitt’s essential immunology. John Wiley & Sons.
- Braden, B. C., & Poljak, R. J. (1995). Structural features of the reactions between antibodies and protein antigens. The FASEB Journal, 9(1), 9-16.
- Gan, S. D., & Patel, K. R. (2013). Enzyme immunoassay and enzyme-linked immunosorbent assay. Journal of Investigative Dermatology, 133(9), e12.
- Crowther, J. R. (2009). The ELISA guidebook. Humana Press.
- Johnstone, A. P., & Turner, M. W. (1997). Antibodies: A practical approach (Vol. 10). Oxford University Press.
- Lequin, R. M. (2005). Enzyme immunoassay (EIA)/enzyme-linked immunosorbent assay (ELISA). Clinical Chemistry, 51(12), 2415-2418.
- Porstmann, T., & Kiessig, S. T. (1992). Enzyme immunoassay techniques: an overview. Journal of Immunological Methods, 150(1-2), 5-21.
- Engvall, E., & Perlmann, P. (1971). Enzyme-linked immunosorbent assay (ELISA) quantitative assay of immunoglobulin G. Immunochemistry, 8(9), 871-874.
- Conradie, J. D., Govender, M., & Müller, E. E. (1997). ABTS, an ideal chromogenic substrate for the detection of peroxidase-labeled probes in microplate enzyme immunoassays. Journal of Immunoassay, 18(2), 151-164.
- Findlay, J. W., Smith, W. C., Lee, J. W., Nordblom, G. D., Das, I., DeSilva, B. S., & Bowsher, R. R. (2000). Validation of immunoassays for bioanalysis: a pharmaceutical industry perspective. Journal of Pharmaceutical and Biomedical Analysis, 21(6), 1249-1273.
- Krone, J., & Friedland, J. (2008). Semi-automated non-instrument-based immunoassay with near-sensor immobilization and homogeneous chemiluminescence detection. Clinical Chemistry, 54(5), 925-933.
- Kemeny, D. M., & Chantler, S. (1988). An introduction to ELISA. In ELISA and Other Solid Phase Immunoassays (pp. 9-26). John Wiley & Sons Ltd.
- Vitzthum, F., Behrens, F., Anderson, N. L., & Shaw, J. H. (2005). Proteomics: from basic research to diagnostic applications. A review of requirements & needs. Journal of Proteome Research, 4(4), 1086-1097.
- Hornbeck, P. V. (1992). Enzyme-linked immunosorbent assays. Current Protocols in Immunology, 2.1. 1-2.1. 22.
- Bjerrum, O. J., & Schafer-Nielsen, C. (1986). Analytical general procedure. In Analytical Use of Enzyme-Linked Immunosorbent Assays (ELISA): An Introduction (pp. 23-56). Springer, Dordrecht.
- Badawy, A. A. B., Morgan, C. J., & Turner, J. A. (2008). Application of the Phenomenex EZ: fasten™ and Ab18G biosensor surfaces to improve drug-protein binding assays. Biosensors, 5(1), 286-311.
- Kelso, D. M. (1994). Enzyme immunoassay data processing. Current Opinion in Biotechnology, 5(1), 39-44.
- Crowther, J. R. (2009). The ELISA guidebook. Humana Press.
- Nisnevitch, M., & Firer, M. A. (2001). The solid phase in affinity chromatography. Journal of Biochemical and Biophysical Methods, 49(1-3), 467-480.
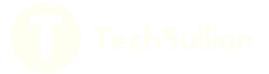