The growing complexity of semiconductor technology has introduced formidable challenges in achieving timing closure for high-frequency designs. As advanced nodes push beyond 1 GHz, physical and logical constraints require new approaches to maintain efficiency. Rajesh Arsid, a researcher focused on semiconductor advancements, explores strategic methodologies to address these pressing issues.
The Growing Challenges of Modern RTL Design
With shrinking technology nodes below 7nm, implementation teams face increasing interconnect delays, higher capacitive loading, and timing uncertainties. The evolution of RTL designs has led to deeper logical dependencies, making traditional timing closure techniques inadequate. The shift from 28nm to 7nm has resulted in a significant increase in interconnect delays, amplifying the need for innovative solutions. Additionally, the reliance on FinFET and Gate-All-Around technologies has introduced factors such as electrostatic interference and power density concerns, which complicate the timing closure process.
Cross-Functional Collaboration for Efficient Implementation
Achieving timing closure requires collaboration between front-end RTL designers and back-end physical implementation teams. Research indicates that structured handoff processes and early design reviews can reduce critical path violations. Engaging cross-functional teams early in the design phase ensures that potential bottlenecks are identified before they manifest in physical implementation. Additionally, automated design validation tools and AI-driven predictive models are being integrated into workflows to enhance efficiency and reduce errors before the physical design stage begins.
Advanced Netlist Optimization for Enhanced Performance
Moving beyond conventional synthesis techniques, multi-corner optimization is proving to be an effective method in high-frequency designs. Studies show that analyzing designs across multiple process, voltage, and temperature (PVT) corners reduces timing violations during initial place-and-route stages. Moreover, targeted critical path restructuring improves high-fan-out nets, enhancing overall circuit reliability. With machine learning-based netlist analysis, designers can now identify weak points in timing structures and preemptively apply targeted optimizations, reducing the number of closure iterations required.
Strategic Floorplanning to Minimize Routing Congestion
As designs grow in complexity, efficient floorplanning becomes critical. A well-structured physical layout aligned with logical design structures can reduce wire length by up to 25%. Timing-aware placement strategies ensure that critical paths are optimized, leading to a reduction in maximum path delays. Furthermore, integrating power grid optimization techniques mitigates IR drop effects, enhancing timing stability. The latest advancements in 3D-IC technology allow for more efficient stacking of logic elements, reducing interconnect latency and improving power distribution across the design.
Innovative Clock Tree Synthesis for Stability
Clock tree methodologies play a vital role in timing closure, especially in high-frequency designs. Research suggests that balancing clock distribution structures can reduce global skew. Implementing multi-corner clock tree synthesis ensures minimal performance variation across different environmental conditions. Additionally, local skew management techniques help improve timing margins, leading to a more predictable implementation process. Recent developments in dynamic clock gating techniques allow for better power management while preserving timing integrity, making modern clock trees both efficient and robust.
Congestion-Aware Routing Strategies
Routing congestion can significantly impact signal integrity and overall performance. Early congestion analysis has been shown to reduce routing-induced timing violations. Strategic layer assignment for critical nets minimizes RC delays, while shielding techniques protect noise-sensitive paths, preventing crosstalk-induced delays. These methodologies enhance design predictability and reduce the number of late-stage iterations. The use of AI-driven congestion prediction tools is further refining routing optimization, ensuring smoother physical implementation and reducing the risk of late-stage design failures.
The Future of High-Performance Designs
The integration of these advanced methodologies has demonstrated measurable performance improvements in semiconductor designs. From AI accelerators to high-performance computing (HPC) systems, adopting structured timing closure techniques enables higher computational throughput and increased operating frequencies. As the semiconductor industry evolves, these strategic approaches will be pivotal in shaping the next generation of high-performance silicon designs. Additionally, the rise of chiplet-based architectures is driving new methodologies for partitioning designs across multiple smaller chips, allowing for better modularity, efficiency, and scalability in complex computing applications.
In conclusion, as semiconductor designs grow in complexity, advanced timing closure strategies are essential. By integrating cross-functional collaboration, netlist optimization, and congestion-aware routing, engineers can ensure high-frequency performance. Rajesh Arsid’s insights highlight the importance of these methodologies in shaping the next generation of computing solutions.
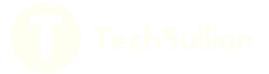