An overview of the latest in next-generation sequencing techniques and technologies.
Next-generation sequencing (NGS), or ‘high-throughput sequencing’, is a catch-all term that refers to various modern sequencing techniques. These techniques enable the rapid sequencing of the base pairs in DNA and RNA samples, which is essential to genetic research and discovery. Next-generation sequencing technologies support applications like gene expression profiling, molecular analysis, and detection of epigenetic changes, making them essential to advancing treatments for genetic conditions, tackling obesity, and even enhancing COVID-19 testing.
BioTechniques, the international journal of life science methods, closely follows ongoing advancements in next-generation sequencing and publishes the latest updates to the techniques’ workflows and methods in its peer-reviewed, open-access journal. BioTechniques supports this with news of next-generation sequencing developments and applications in its online multimedia website.
Here’s an overview of the progress that next-generation sequencing has seen over recent years and the developments we can expect to see in the future.
How Automated Sequencing Can Improve Genomic Profiling
Genomic profiling is an important tool in cancer treatments and the study of oncology and other disease areas. The profiling technique involves reading the sequence of genetic materials from any organism, whether human, animal, bacteria, or virus. It’s possible to either read a complete sequence of a genome or profile the parts of genomes that are relevant to a particular disease.
Now, automated sequencing can accelerate the genomic profiling process, allowing clinicians to generate and access profiles rapidly – improving reproducibility in research.
Using Genomic Profiling to Identify Disease-Causing Mutations
Cancer is a metabolic disease that is rooted in DNA. The disease is often caused by changes in genetic material that alter protein expression or the types of proteins (known as oncogenic drivers). These proteins skew cell mechanisms and cause them to divide. Genomic profiling identifies how a patient’s DNA has changed and which therapies will best support them.
Researchers compare genomic profiles against ‘reference human sequences’ to identify shifts in genetic material. When it comes to inherited disease, researchers can also sequence genomic changes that a patient has inherited from their parents or de novo mutations that have been present since birth. They can examine the changes in the DNA to identify the cause of an inherited disease.
The Evolution of Genomic Profiling Technologies
The first next-generation sequencing technology used to conduct genomic profiling was Sanger sequencing technology, which was based on slab gel electrophoresis platforms. Then, the first human genome project was performed using capillary electrophoresis platforms.
In the mid noughties, the next generation of sequencing technologies emerged. These technologies enabled researchers to complete millions of sequencing reads simultaneously (instead of the tens or hundreds that were possible beforehand). This lowered the cost of sequencing and genomic profiling, making it possible to apply the techniques in many more areas.
The Latest Developments in Genomic Profiling
Advancements in the automation of next-generation sequencing have progressed genomic profiling over recent years. For example, systems like the Ion Torrent Genexus System automate both DNA sequencing processes and the processes that researchers complete before sequence generation. This automation removes many sources of error by minimizing the number of steps in the process.
The Ion Torrent Genexus System is currently only available for research use. But the system is now undergoing the FDA approval process to make it available for clinical use. Automation of the library preparation, template preparation, and sequencing in a single platform will reduce the time required to complete testing processes. In turn, the generation of results will also become quicker, solving long delays that cause anxiety for patients, oncologists, and pathologists – especially in cancer care.
We can expect to see more widespread usage of and recognition for genomic profiling over the next few years, enabling more distributed hospital environments.
The Impact of Next-Generation Sequencing on Cancer Research
Next-generation sequencing has been essential to the advancement of cancer research over the past decade, originally in targeted therapies. This is because next-generation sequencing can identify the driving mutation of a patient’s cancer and identify which therapy will be most effective. Over recent years, next-generation sequencing has also formed the basis for personalized immunotherapy.
Although next-generation sequencing technologies haven’t evolved hugely over the past few years, these technologies’ uses and applications have. For example, next-generation sequencing for liquid biopsies can help researchers achieve non-invasive assays for patients. These assays can determine a high level of information and have a minimal negative impact on patients. This means that the technology has few limitations.
Instead, it’s the physical limits of samples that raise challenges. However, there are new solutions in the pipelines to address these challenges. The other side of the difficulty is that there are often only so many mutant copies present. As next-generation sequencing evolves, researchers will analyze more regions across a genome, allowing them to analyze more broadly (especially as sequencing techniques get cheaper). This will be essential for the progression of cancer research.
Over the next 5–10 years, we can expect next-generation sequencing to become more prominent in the clinical space. Sequencing technologies should also advance to determine higher-quality information, such as longer reads.
Whole Genome Sequencing and Difficult-to-Diagnose Disorders
Cancer aside, many conditions – especially genetic disorders – manifest in several ways, making them difficult to diagnose. As a result, many patients go through several referrals and tests before practitioners can identify what their condition is. It can take years to get a diagnosis, which can be particularly challenging in the genetic space, especially when it comes to diagnosing rare diseases or diagnosing multiple diseases at the same time. However, it’s often essential to get a diagnosis quickly. This is especially true for some young children who need treatment almost immediately to prevent permanent brain damage or to save their lives.
Whole genome sequencing is key to reaching these diagnoses. Two techniques aid whole genome sequencing:
- Phenotyping: identifying and recording the symptoms of a patient’s condition.
- Genotyping: determining differences in an individual’s genetic makeup by analyzing their DNA sequence using biological assays.
As technologies advance, we can hope to see these techniques progress so that practitioners can diagnose disorders more easily. We will also soon see the combination of short- and long-read sequencing so patients can undergo both, which should overcome the limitations of each. While long-read sequencing reads long fragments of DNA (between 5,000 and 30,000 base pairs), short-read sequencing reads tiny fragments of DNA. Longer reads are easier to process and analyze, but short reads achieve higher coverage.
In the meantime, diagnoses for all kinds of conditions are becoming more accessible, not only because diagnostics are becoming quicker and more cost-effective, but also because of the rise of telehealth (especially amid the COVID-19 pandemic). When it comes to genetic conditions, this is particularly helpful for those who aren’t within easy reach of genetic departments and genetic counselors.
Completing the Human Genome Sequence
As sequencing techniques evolve, new technology has now enabled researchers to complete the human genome sequence (except for the Y chromosome). Since the first draft of the human genome sequence was published in 2001, emerging technologies have advanced its resolution. The most recent version has been used as a reference since 2013 and was patched in 2019. However, this version is missing 8% of the sequence because of difficulties to do with sequencing complicated sections.
In summer 2021, the Telomere-to-Telomere (T2T) Consortium published a currently un-peer-reviewed preprint, announcing the addition of 115 protein-coding genes and 200 million DNA base pairs to the human genome sequence. This raises the totals to 19,969 protein-coding genes (a 0.4% increase) and 3.05 billion base pairs (a 4.5% increase). The new sequence, T2T-CHM13, was made possible by merging the benefits of new competing long-read technologies from Oxford Nanopore and Pacific Biosciences. This increased the length of DNA that is accurately scannable at one time from a few hundred base pairs to 20,000. The longer the fragments of DNA, the easier they are to put together correctly.
As the team used a cell line from a hydatidiform mole (formed by inseminating an egg with no nucleus), they didn’t need to distinguish chromosomes from two individuals. However, as the sperm used carried an X chromosome, the new sequence doesn’t cover the Y chromosome. Plans to work on the Y chromosome and sequence genomes containing chromosomes from two parents are now in progress.
Target Enrichment for Next-Generation Sequencing
Rather than sequencing straight from a sample, researchers usually undertake target enrichment before getting started with next-generation sequencing. This is because researchers are usually only interested in sequencing particular regions of a genome. The entire human genome is approximately three billion bases, so sequencing select regions is much more cost-effective and time efficient than sequencing a whole genome. Sequencing a particular region of a genome also allows researchers to conduct a deeper analysis of that sample and its applications.
Technologies for Target Enrichment
There are two main types of technologies that achieve target enrichment. The first is amplicon technology (which many individuals think of as PCR products). Amplicon technologies target specific primers to amplify regions of a genome. Researchers usually focus on hundreds or even thousands of amplicon targets. The second type of technology is based on hybridization capture, which usually focuses on broad targets. These technologies are highly efficient and enable even coverage in enrichment. Therefore, hybridization technology is more about performance, and amplicon technology is more about ease of use and turnaround time.
The Challenges of Amplicon and Hybridization Capture Technologies
Although amplicon technology is faster and easier to implement than hybridization technology, it doesn’t achieve as much evenness of coverage. The amplicons in a library might not all amplify as well as each other. Researchers tend to see more of some amplicons and fewer of others. The more amplicons in the library, the bigger this problem can be.
Secondly, researchers need to know both the forward primer and the reverse primer binding sites. When a researcher has a structural rearrangement but doesn’t know what it is, they can’t assign a primer to it. Therefore, amplicon-based enrichment can only be used for DNA targets that have well-documented sequences. This limits the use of these techniques in next-generation sequencing discovery studies or variant novel identification. Plus, every library molecule contains primers. But researchers don’t need to sequence primers to interrogate bases, so they need to trim primers off.
On the other hand, hybridization capture technology typically involves a more complicated workflow. The process starts with library preparation: researchers fragment genomic DNA and make library molecules. They make specific probes to the regions of interest and hybridize these probes to the regions of interest. But the hybridization has to take place overnight – or longer – to capture all targets effectively. The multiple steps and time required can be cumbersome.
New Technologies to Overcome Challenges
The risk of biases and uneven coverage grows the more amplification cycles a researcher completes. Therefore, researchers need to reduce the number of amplification cycles to overcome challenges in amplicon technologies. Meanwhile, in hybridization capture technologies, improvements involve optimizing buffers for the overnight hybridization capture to make this process shorter. New technologies can replace the hybridization step with a primer extension step, which is quicker, easier to use, and enables better performance.
Researchers in the oncology field must choose carefully between amplicon and hybridization capture technologies for their practices. New tools can help them decide between fast and easy workflows and high-performing workflows, even for challenging samples.
Other Developments in Next-Generation Sequencing
As next-generation sequencing advances, emerging techniques could prove key to new areas, such as tackling obesity. New research from the University of Virginia’s College of Arts and Sciences has added a significant contribution to the growing evidence that lifestyle choices aren’t solely responsible for obesity: our genes can be responsible too. The regulation of fat storage, the ways that the body converts food into fuel, and the genes that control these functions can also contribute to obesity.
The new research, led by Eyleen O’Rourke, supports multiple genome-wide association studies (GWAS) that have discovered hundreds of genes associated with obesity. O’Rourke’s study tested 293 genes, each associated with obesity. Researchers screened the genes in two groups, one group following a regular diet and the other following a high-fructose diet.
The study concluded that 17 of the genes studied affect obesity, identified 14 genes that cause obesity, and identified 3 genes that can help prevent obesity. These findings, resulting from next-generation sequencing techniques, have provided a list of causative genes for more detailed analysis in higher-order models. Such analysis could uncover potential therapeutic targets for anti-obesity treatments.
Meanwhile, a new, state-of-the-art sequencing facility has enhanced COVID-19 testing in Nigeria. This has stepped up the genetics infrastructure in Africa, using several technologies to power various applications like whole-exome sequencing, whole-genome sequencing, and genotyping. This progression will generate quality data from samples and aid the equalization of precision medicine in Africa.
About BioTechniques
Since BioTechniques’ inception in 1983, the leading journal has published an ongoing collection of the latest methods and techniques in scientific and medical advancements. Rather than focusing on treatments, BioTechniques highlights the methods and techniques – and the reproducibility of these – that make treatments possible. Some of these methods include polymerase chain reaction (PCR), western blotting, CRISPR gene editing, chromatography, and next-generation sequencing.
BioTechniques also curates and shares updates in methods and techniques on its online platform. Here, scientists and lab workers at all levels find a wealth of articles, videos, interviews, eBooks, webinars, and podcasts (like this Talking Techniques episode on target enrichment for next-generation sequencing) to further their knowledge, both in life science fields and related disciplines.
Learn more about BioTechniques.
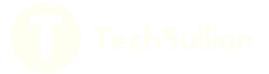