A groundbreaking revolution is underway, promising to reshape the landscape of computing as we know it. Quantum electronics, and more specifically, quantum computing, has emerged as a frontier that holds the potential to solve complex problems that traditional computers struggle with. In this article, we embark on a journey to demystify the future of quantum electronics, providing a deep dive into the fascinating world of quantum computing.
Understanding the Quantum Difference:
To comprehend the future of quantum electronics, it’s essential to grasp the fundamental differences between classical and quantum computing. Classical computers, the ones we use in our daily lives, operate based on bits—units of information that exist in a binary state, either 0 or 1. Quantum computers, on the other hand, leverage quantum bits, or qubits, which can exist in multiple states simultaneously, thanks to the principles of superposition and entanglement.
Superposition and Quantum Parallelism:
Quantum superposition allows qubits to exist in multiple states at once, exponentially increasing the computational power of quantum computers. This phenomenon enables these systems to perform multiple calculations simultaneously, a concept known as quantum parallelism. In contrast, classical computers can only perform one calculation at a time, making quantum computers potentially exponentially faster for certain types of problems.
Entanglement:
The Quantum Connection:
Entanglement is another intriguing quantum phenomenon that plays a crucial role in the power of quantum computing. When qubits become entangled, the state of one qubit directly influences the state of another, regardless of the distance between them. This interconnectedness allows for the creation of quantum systems that can process information in ways that classical computers simply cannot.
Quantum Bits vs Classical Bits:
The Power of Exponential Growth:
In classical computing, adding more bits increases the capacity linearly. However, in quantum computing, the addition of qubits leads to exponential growth in computational capacity. A quantum computer with a relatively small number of qubits has the potential to outperform even the most powerful classical supercomputers for specific tasks, such as factoring large numbers or simulating quantum physics.
Applications of Quantum Computing:
Unraveling Complex Problems:
The future of quantum electronics holds immense promise for solving complex problems that were once thought to be insurmountable. Quantum computers excel in areas such as cryptography, optimization, and simulation of quantum systems. For instance, they can potentially break widely-used encryption algorithms, simulate molecular structures for drug discovery, and optimize complex logistical challenges.
Quantum Supremacy:
A Milestone Achievement:
Quantum supremacy is a term that signifies the point at which a quantum computer can outperform the most advanced classical computers for a specific task. In 2019, Google claimed to achieve quantum supremacy with its 53-qubit Sycamore processor, solving a particular problem faster than the world’s most powerful supercomputers could. This milestone marked a pivotal moment in the trajectory of quantum electronics, showcasing the tangible capabilities of quantum computing.
Challenges on the Quantum Horizon:
While the future of quantum electronics is bright, it is not without its challenges. Quantum computers are highly sensitive to their environment, requiring extremely low temperatures to operate. The phenomenon of decoherence, where quantum information is lost due to interactions with the environment, poses a significant hurdle. Researchers are actively exploring ways to mitigate these challenges and make quantum computers more robust.
Quantum Hardware:
Building the Foundations:
The development of quantum computers relies heavily on the progress made in quantum hardware. Various technologies, including superconducting circuits, trapped ions, and topological qubits, are being explored as potential candidates for building scalable and error-resistant quantum processors. Advancements in quantum hardware are pivotal for realizing the full potential of quantum computing.
Quantum Software:
Navigating the Quantum Landscape:
In tandem with hardware, the evolution of quantum software is crucial for unlocking the capabilities of quantum computers. Programming languages and algorithms tailored for quantum computing are actively being developed. As quantum computers become more accessible, a new generation of software engineers will play a pivotal role in harnessing the power of quantum algorithms to address real-world problems.
Quantum Networking:
Connecting the Quantum Dots:
The future of quantum electronics extends beyond individual quantum computers. Quantum networking aims to create a quantum internet that enables the transmission of quantum information over long distances. Quantum key distribution, a method for secure communication, is one of the promising applications of quantum networking. This advancement holds the potential to revolutionize secure communication in the digital age.
Ethical Considerations in Quantum Computing:
As the quantum revolution unfolds, ethical considerations come to the forefront. The ability of quantum computers to break existing encryption methods raises concerns about data security. Ethical guidelines and regulations are essential to ensure responsible development and deployment of quantum technologies. Striking a balance between innovation and ethical considerations will be critical in navigating the quantum landscape.
The Quantum Workforce:
Bridging the Knowledge Gap:
The realization of quantum computing’s potential requires a skilled workforce equipped with the knowledge to develop, program, and maintain quantum systems. Educational initiatives and industry collaborations are essential in bridging the knowledge gap and preparing the next generation of quantum scientists, engineers, and programmers.
Conclusion:
The future of quantum electronics, particularly quantum computing, holds immense promise and potential. The ability to process information at a quantum level opens doors to solving complex problems that were once thought to be beyond the reach of classical computers. While challenges exist, the trajectory of quantum electronics is one of relentless innovation and exploration. As researchers, engineers, and enthusiasts continue to navigate the quantum frontier, we find ourselves on the brink of a new era in computing—one where the rules of classical physics no longer limit the boundless possibilities of quantum electronics.
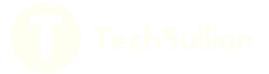